Ep 119: Dr Aneeqa Khan "Fusion Energy: The Materials Challenge"
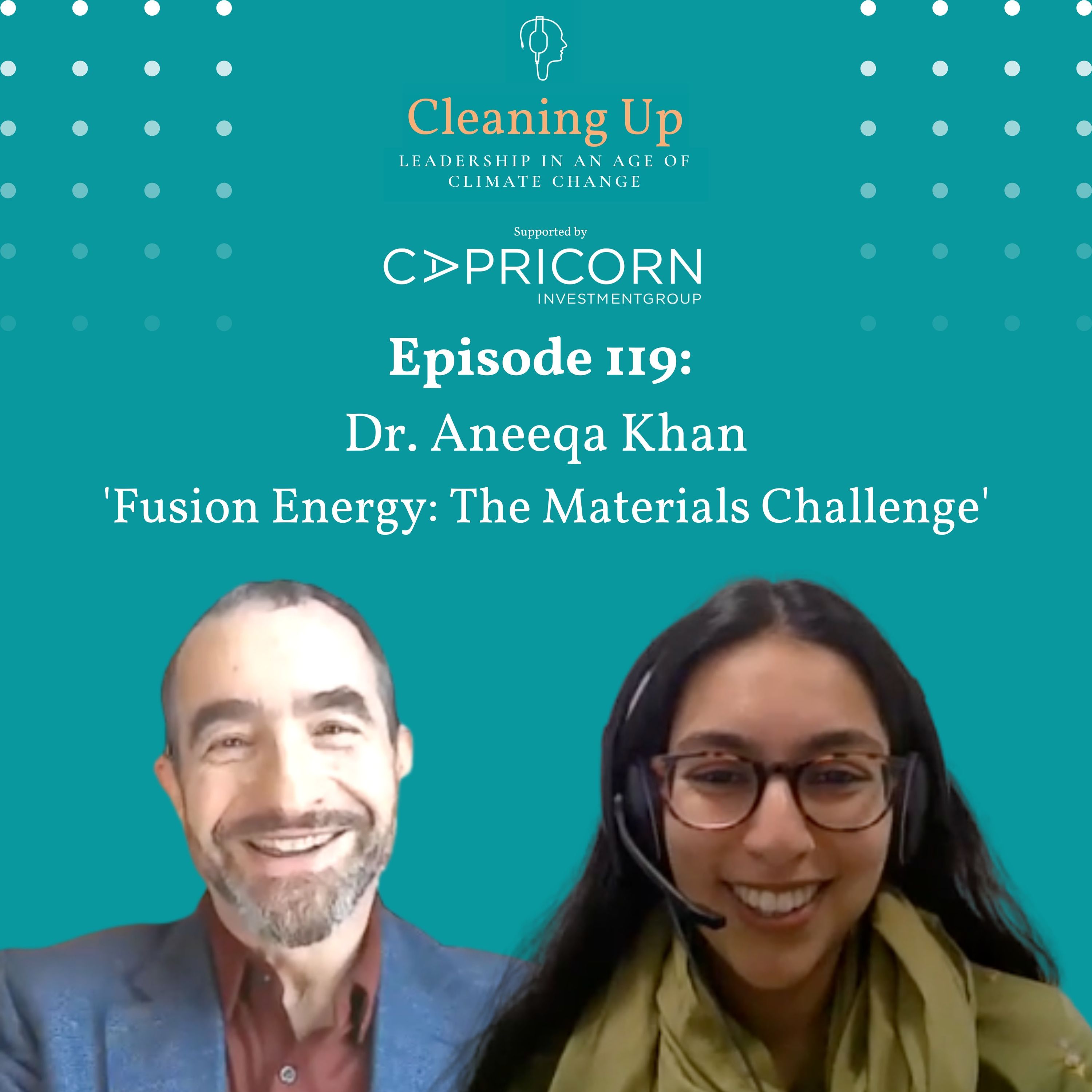
This week on Cleaning Up, Michael welcomes Dr Aneeqa Khan, Manchester-ISIS Neutron and Muon Source Research Fellow in Nuclear Fusion.
Aneeqa had insights to offer on the significance of the recent fusion breakthrough at Lawrence Livermore National Laboratory, some of the challenges facing nuclear scientists and engineers, and the race to bring a raft of fusion technologies to commercialisation and grid integration.
Edited Highlights: CLICK HERE
Links and Related Episodes:
Dr. Khan spoke to the New Statesman about fusion and the climate: CLICK HERE
Discover Cleaning Up’s Nuclear playlist on YouTube, including Episode 29 with Steven Chu: CLICK HERE
Dr. Khan’s recent research output can be found here: CLICK HERE
Learn about Helion’s plasma accelerator: CLICK HERE
Cleaning Up Episode 114 featured James DeMuth, CEO of Seurat Technologies: CLICK HERE
Guest Bio
Dr Khan has been Manchester-ISIS Neutron and Muon Source Research Fellow in Nuclear Fusion at the University of Manchester since 2019. Previously, she’s held postdoctoral positions at the Max Planck Institute for Plasma Physics in Garching, Germany, and at ITER Organization in Saint-Paul-lès-Durance, France.
Dr Khan holds a BEng in Mechanical Engineering and a Ph.D. in nuclear engineering from the University of Manchester. Dr Khan’s areas of research interest include material deposition, irradiation effects and fuel retention.
Michael Liebreich So Aneeqa, thank you so much for joining us here on Cleaning Up.
Aneeqa Khan Thanks, Michael. I'm really looking forward to it.
ML Now, are you calling in from somewhere in Manchester, would that be right?
AK Yeah, I'm in Manchester University at the moment.
ML Fabulous. Now the way that we usually start is by… I'd like to ask you to describe what you do in your own words, because I'll mangle it and get it wrong if I try. So, who are you, and what is it that you do?
AK So yeah, I'm Aneeqa Khan. I'm a research fellow based at the University of Manchester, which means I do research and my area of research is nuclear fusion. So, I specialize in materials and engineering for fusion applications. I can talk more about like the day to day what that means?
ML That will probably become apparent as we go through, because obviously, what I want to do is to do a fairly broad overview of fusion to start with. Because I've watched some of the things that you talk about, and you get into fabulous levels of detail about vapour deposition of diamonds, and I do want to get into that. But maybe we should just take a step back to start with, and give an overview of fusion. It's been in the news, obviously, because of this breakthrough at the end of last year, at the Lawrence Livermore Laboratory, which was producing more energy out, than in. Just very big picture, in your field, how momentous or otherwise was that breakthrough?
AK So for fusion science, that’s huge, that's never been done before. We've never had a reaction where more energy is put into the reaction than we get out. So, massive breakthrough, and huge congratulations to all the scientists working on it. In terms of having fusion as a source of energy or a source of electricity, we're still a very long way off, because that energy out doesn't take into account all the energy the lasers took, for example, to heat up the reaction that occurred there. So, although there was a net energy gain of the reaction itself, engineering-wise, there wasn't an energy gain. So, a long way to go in terms of fusion as an electricity source.
ML That's really at the heart, I think, of where… For my audience, which is… there will be some pure scientists listening, but there will also be a lot of just generalists, there's a lot of energy industry folks, a lot of regulators, a lot of civic society, climate change people and so on. And so, we're trying to figure out, how important and how close is it? And I look at it, and I say, so that laser was two megajoules of laser, and it produced three megajoules out. So, that's incredible. But the laser took 300 megajoules to create. And of course, what it put out was just heat. So, if you then tried to generate electricity from it, you'd be getting out 1% of what you need to create the lasers in the first place.
AK Yeah, absolutely. No, that's a completely correct understanding of where we are at the moment in terms of that technology.
ML Okay, so it's 100x improvement needed overall, just to break even; not to produce net electricity, but just to keep the whole thing going. Is that fair? Or is that harsh?
AK I mean, for inertial confinement fusion, I guess if we look at that one experiment, that's what the data is telling us. But it's not the only type of fusion confinement that is available. So, there's also magnetically-confined fusion, which uses magnets to hold the fusion reaction in place. So, there's a lot of other approaches that are being taken. That's the only example where we've managed to get, where the actual reaction itself has had a net gain. Other techniques haven't shown this yet, but I believe that they will do sooner rather than later. But for sure there's a huge area needed for improving the efficiency all around, whether we're using lasers or using magnets to confine the fusion reaction.
ML Maybe towards the end, let's come back some of the engineering challenges, because it is such a huge topic, and you've done some of my work for me by starting to mention these other ways of confining. But let's come back to those engineering challenges, of how you might get heat or some other useful work out and talk about… Can you start with just… I think we'll assume that the audience knows that you're starting with little atoms and banging them together rather than fission, which is starting with a big one and splitting it apart. So, let's start with that. But there are lots of different ways of doing it. And one of the exciting things about the field - it feels to me as an outsider - is there's just so many different ways, different combinations of deuterium and tritium and helium and boron, and different geometries; it all feels a bit like the Wild West, or the kind of pre-Cambrian explosion of interest and different models. Can we try and break that down, starting either with the geometries, or perhaps even starting with the different nuclear reactions that you're trying to spur?
AK Absolutely. So, as you stated, we start off with two nuclei that we want to fuse together. At the moment, typically, the most common combination is using deuterium and tritium. So, isotopes of hydrogen, that's kind of the most widely-accepted reaction that people want to use. In order for them to fuse together, there's kind of this triple product rule that we need to obey. So, we need to have enough nuclei, that have enough energy, for a long enough time, so that, there needs to be enough of them, they need to have enough energy, and we need to [inaudible] them for as long as possible in order for them to fuse. Typically, to do that, in a magnetically confined fusion device, we need to have them very, very hot; actually, ten times hotter than the centre of the Sun. So, we're talking about temperatures of 150 million degrees Kelvin, in the centre of a plasma. So, this is a super, super heated gas, which causes your atoms to split up into your nuclei, and then your electrons as well.
ML Can I ask a stupid question? Because that's kind of my role here, okay? Why does it have to be ten times as hot as the sun?
AK So in the sun, there's a lot of pressure, it’s huge body of plasma and particles all swirling around. We don't have that same kind of pressure on Earth. So basically, because there's not that pressure, we have to compensate for that by having temperatures hotter than the centre of the Sun.
ML Thank you.
AK No problem. So yeah, very, very extreme temperatures first of all, so it's very, very hot in the centre of your plasma. As I mentioned before, you can use magnets to confine that in a doughnut-shaped device, which is called a tokamak. So, that's one of the ways that we're working, or one of the reactor designs that we're working on at the moment. So, you have this doughnut-shaped device, you have a plasma running around on the inside, and it's confined. And the idea is that in the future, when you generate the heat energy, you can extract that from the device and use it to generate electricity or other applications that require high heat. The tokamak is not the only design, there's also, in magnetically confined fusion, something called a stellarator, which kind of looks like Salvador Dali drew a donut. So, it's this weird, kind of twisted device, which is also in a ring confinement but it’s very, very twisted, and a very particular geometry. And the way I like explain…
ML On the stellarator, just let me say: if anybody who is listening or watching – in the video, who knows, we might even be able to edit a little photograph of a stellarator in there - there's this thing called the Wendelstein 7x stellarator, because it's in Germany. And it just looks like the Deathstar, doesn't it?
AK Yeah, absolutely. It's a crazy, crazy geometry.
ML It's the most extraordinary looking thing. Very difficult to fabricate, I understand that’s the challenge with it.
AK Yeah, so a stellarator is more difficult to fabricate, but actually, it's easier to run. So, it can run for quite an extended period of time. Whereas the tokamak is easier to produce and easier to build, but at the moment, it currently can only run in kind of pulses, due to the nature of the magnets that we're using at the moment.
ML Okay, but then there's also inertial confinement, which is what they did at Lawrence Livermore. And then there's a couple of other ways of doing it, right?
AK Yeah, there's a couple of other ways but I'm not gonna go into too much detail on those because they're quite… I'm not an expert, Magnets are my kind of key area, I can talk a bit about inertial, but the others kind of a bit more unique. There's not that many people working on the other types of fusion, the main ones are really kind of inertial or magnetically confined
ML Okay, and then, coming back to the nuclei that you're choosing. So, deuterium-deuterium has very low yields, as I understand it, and that's why it's just much better to go to deuterium-tritium. But there are others, there's boron, and there's helium and so on. Why deuterium-tritium?
AK So deuterium-tritium basically has the highest likelihood of occurring. So, the highest cross-section compared to similar reactions within the range. So, that's why we go for deuterium-tritium, just because it’s more likely to happen as a fusion reaction.
ML Okay, but then isn't tritium… I mean, we're sort of exploring around some of the tradeoffs already, right? So, you've got the temperature, you don't want to have to go too hot. It's a bit like Goldilocks: you want something that's not too hot, enough pressure, the right temperature, the right yield. We’ll come back to the energies of the particles, or the radiation that's produced. But the tritium; it's not trivial to get hold of tritium, correct?
AK So, currently, our main source of tritium for fusion reactions comes from CANDU reactors, which are fission reactors that produce tritium as a byproduct. So, any tritium that we're using in experimental reactors at the moment tends to come from those. However, the plan is in the future that when we're building fusion reactors, but we can [inaudible] within the system by utilizing the high-energy neutrons that are produced from the deuterium-tritium reaction, and having lithium panels on the inside walls of the reactor that can interact with those neutrons, generating more tritium. So, kind of making this loop where we can generate more fuel whilst also generating energy.
ML Okay, so let's do a collision, let's do the reaction. You've got deuterium and tritium, and you get them hot enough and at high enough pressure, however you do that, and that produces…?
AK That produces 14 mega electron volt neutrons, so very high energy neutrons, and also helium.
ML And also helium. Okay. And those, do they spew out, do they stay there, what happens? Because you talked about the neutron, perhaps, combining with lithium and producing more tritium?
AK Yeah, so those neutrons don't have any charge. So, they're not confined by the magnetic field. So, they can actually impact on the wall of your reactor. And we want to use that to our advantage in the future to produce tritium. But it could also be an issue for materials. because your materials basically have to be able to withstand this load of a very, very high energy neutrons. So, it is something that as material scientists and engineers, we need to be aware of, and developing materials that can kind of withstand or resist that irradiation damage. The helium can also interact with the walls of your reactor as well, so you can get things like bubbles or blisters. You get really crazy effects happening in tungsten, which is one of the materials I'm working on, where you get this nanostructure that forms which is called “fuzz” - very creatively named - and it turns your tungsten black, and you basically get these crazy tendrils on the surface of your tungsten. So…
ML Does that come from the neutron interaction? What's interacting to create that fuzz?
AK The helium, so the helium interacts. Yeah, depending on the temperatures - you have to fulfill a few certain conditions - but yeah, your helium can interact with your tungsten to produce that
ML So it comes out with energy, it penetrates the tungsten, and it sits there, and then it's creating this fuzz?
AK Yeah, so the mechanism is not fully understood. It’s a very active area of research at the moment, but we believe that we get these kinds of bubbles which burst, and this produces this kind of microstructure.
ML So, when I spoke to, on Cleaning Up, Steven Chu, who was the Nobel laureate, who was also Secretary of Energy under Barack Obama, the first of his two Secretaries of Energy… And I asked him about fusion, and he basically - I should have gone back and looked for the exact quote - but he kind of said, good luck, because the linings of these things are going to have to be replaced just constantly. So, you've got this constant maintenance challenge. Is it also going to become radioactive? The tritium is also going to be embedded in the lining, is it not going to be radioactive?
AK Well, the tritium is actually not… that's got a very short half-life, so even if we do have tritium embedded; it's not ideal due to regulations, how much tritium you're allowed at the moment in facilities or reactors. And there is research in how to kind of limit that or how to get the tritium out of components. But the tritium is not that much of a worry, but the 14 MeV neutrons interacting with your materials could generate significant amounts of waste. It would be low-level waste, in comparison to traditional fission reactors, but the volume could potentially be more if we're not using the right materials, or using kind of reduced-activation type of materials.
ML So the neutron is creating a kind of soup of different things; as it gives up its energy, it's just creating all sorts of stuff in the in the walls of the tokomak.
AK Exactly. So, it can interact with your materials, cause transportation, cause displacement… Kind of like a snooker ball interacting with other snooker balls, if you can picture it that way.
ML Okay, and what about the non-tokomak designs? Do they have the same sort of…Because we're going to come on to your area of specialism, the materials… But somebody listening to this could say, well, yeah, but all of this is irrelevant, because it's going to be some different model, either an inertial confinement or a gravitational confinement, or one of these non-ignition, where you create some plasma in two different parts of the machine, and then you ram it together. So, all of this is going to be irrelevant. What's your response to that?
AK I mean, if we're using deuterium and tritium, I think regardless, we're gonna have high temperatures, we're gonna have high-energy neutrons, we're gonna have helium being produced. So, I think these challenges are universal, regardless of what kind of device we're using to confine the reactions.
ML But some people are trying… So, you've got, I think it's TAE, and HB11, or some other companies that are saying no, no, we're going to do deuterium-boron, because that doesn't produce the high-energy neutron, is that right?
AK Yeah, I believe so; I don't know too much about their techniques, or the reactions that they're using. So, I can't speak on that. But yeah, in theory, they could potentially use a different reaction that doesn't produce the same energy of neutrons, for example.
ML And would that solve a lot of the materials challenges, because those nuclear reactions…
AK Yeah, I mean, if the neutrons were lower energy, that would help with materials challenges, but you're still gonna have high temperatures, and I presume other kinds of reactants will be present or other products will be produced that might have their own issues as well. So, I guess it's just choosing what strategy can deal with that.
ML Actually, I'm looking at my crib sheet here: deuterium-helium three, and then you need 600 million Kelvin instead of 150 million. So presumably, you get a whole different set of issues that arise from that?
AK Yeah, and I guess, so even when we say 150 million Kelvin, that's in the centre of our plasma. When we get to the edge of the reactor, it's not 150 million Kelvin, it’s going to be more of the order of 1000 or 2000 degrees, something along those lines. So, whether it's 150 million in the middle, or 600 million, I think that the temperatures at the edge of the reactor will be quite similar, I guess.
ML A 100,000 Kelvin, here or there, who's counting?
AK When you get to those levels, I think it's difficult, right, either way.
ML Although it must have an implication for the amount of energy that you need to supply to achieve ignition?
AK Yeah, I guess. Yeah, to start with, if you don't need to heat it up as much you don't need as much energy to go in. But that is one thing that people want to be moving as we go forward: that we can have of a burning plasma, or plasma that of keep going itself, and sustain itself as well. So, that's another big challenge people are working on.
ML So, let's come on to those material challenges. Because, plus or minus, I think we can agree that all of these different architectures are operating at some edge case limit, that either can or can't be achieved with alloys, or with composites, or with materials that we can envisage. So, you've got this kind of diagram, which I've seen you use in the past, which shows why this is so difficult. I mean, that Goldilocks problem, it's not just too hot or too cold, there's all sorts of things that you're trying to optimize for, correct? Or find a sweet spot for?
AK Yeah, absolutely. Even one of these challenges by themselves would be a huge, huge area for the fusion community to work on, but we're dealing with several ones. So, we've got the 14 MeV neutrons, which I've mentioned already. So, they're impacting your material and causing irradiation damage. Then we've got the extremely high heat loads, as well, in the reactor. There's some really nice slides that I've got where it shows the typical power load in the divertor region of a tokamak, which is kind of like an exhaust. So, you're getting high heat loads there. During normal operations, predicted for ITER which is this tokamak being built in the south of France, you're already exceeding heat loads in a Rolls Royce Trent, a reentry vehicle coming from space, heavy-water reactors; so very, very high heat loads. And then you can have things like disruptions as well, where you're having heat loads which are higher than a rocket going into space.
ML Let’s take a second to talk about what is heat load, for people who are not of an engineering or physics background? I mean, are we talking about a pulse of heat? Are we talking about a gradient between a hot bit and a cold bit, what are we talking about here?
AK Actually both within a tokamak. So, the plasma will generate heat, and that's going to impact energy on a certain area in your reactors, so that's just the heat load that we're talking about. But another crazy statistic about tokamaks is that you've got super-cooled superconductors that are helping generate these magnetic fields, which are cooled to about four Kelvin, so almost absolute zero. And then you've got the centre of the plasma, which is 150 million degrees. In the space of a couple of metres, you're going from almost absolute zero to ten times hotter than the centre of the Sun. And there's nowhere else in the universe with such an intense temperature gradient. So, I think that really sums up the extreme challenges of the fusion reactor.
ML Basically, we're trying to go ten times as hot as the sun, and then we're going to try and get temperatures that are ten times hotter, all the way to almost outer space temperatures; oh, but we're going to try and do it in a couple of meters, in the south of France. Well, it's a nice place to hang out while you’re trying. Although, you are in Manchester, which maybe has not got quite the same cache. But I understand the challenge, there we go.
AK Yeah, so very, very extreme temperatures on either side, hot and cold. Also, as a fusion community, we’ve kind of decided to use reduced activation materials, so materials that don't produce as much radioactive waste, which limits our choices on the periodic table of what we can use. So, that's another consideration. We've also got this helium that I've mentioned, how that can interact with your materials. But the plasma itself, in the reactor, how that interacts with your materials, does it cause erosion. Is it transferring material around the reactor as well, which is depositing it elsewhere? So, that's another huge area of research as well. Then, if we're talking about magnetically-confined fusion, we've got the technology to produce those magnetic fields… I think ITER can go up to 13 Tesla, I think is the magnetic field it’s generating, which if you compare to an MRI scanner in a hospital, which is already very, very high, I think one and a half Tesla. So again, putting into perspective the real extremes that we're requiring. There's also been research into the high-temperature superconductors as well, which could potentially make reactors a lot smaller. They're not actually very high temperature, I think it's around 70-ddKelvin. But it's a bit higher than almost absolute zero. So, that's another area of research that people are working on as well. So, it's the material challenges to produce those magnets, but also, the effect of those very high magnetic fields on the other materials that we're using, as well. We've got tritium, which you've already mentioned as well, if that will get trapped in your material. We don't want it to get trapped, but if it does get trapped, how do we get it out? How do we generate the tritium? What materials do we need for that? That's another huge one. And then kind of like any reactor, where you've got coolants, and things like that, corrosion is always a big issue as well. So, those are the main materials challenges, but as you said, it's finding those solutions that take into account all of them, and understanding all of them together is really difficult.
ML And this, now we're talking about the lining, the bit that's – I don’t know if it’s in contact with the plasma, but it's certainly facing the plasma - but that isn't just a uniform lining? I mean, there's stuff in there, right? There's got to be ways of injecting power, ways of getting power out, there's got to be sensors, there's got to be ports and various pieces of equipment. So, this is not just a question of finding a single material, is it? It's also got to be fabricated and all sorts of challenges, no?
AK Yeah, absolutely. So, there's loads of different materials all working together. And even within a single component on the wall, there'll be interfaces between different materials as well. And so, figuring out how to join those together is a huge engineering problem. Also, as you mentioned, ways of manufacturing; we're talking about very complex geometries. So, sometimes traditional manufacturing works and we can produce the components we need to. But there's a lot of research in additive manufacturing, for example, other kind of novel welding techniques so that we can produce these very complex geometries. But one of the challenges is to kind of keep the same material properties that conventional techniques have used, or have produced as well in the past.
ML We had Episode 114, we had James DeMuth, who has left the Lawrence Livermore Lab, actually the Ignition Facility at Lawrence Livermore, because he spotted that one of the big problems was fabrication, but one of the big opportunities was to use the same lasers – that's the lasers in an inertial confinement lab - and he's taking those lasers and he's actually using it for additive manufacturing. Because the components that you're going to need could be, I think he said, five or six metres across, Even for laser inertial confinement, presumably even for tokamaks, right now, it's even bigger than that?
AK Yeah, it really varies. So, we have some kind of things that with very, very small components that are built up together to make the larger ones as well, or you have very large components as well. But absolutely, there's so much transfer between different industries, as well as, that’s a huge area of research. And manufacturing. and developing those supply chains; supply chains is something which I think is really starting to pick up now. When I entered fusion, it was very much research-driven and we were producing very, very small amounts of materials, materials in labs and things like that. That's still happening for the low technology readiness level materials, but there's now been a real kind of shift of, how can we produce these materials on mass on a on a large scale? Because producing a kilo, or two kilos, is not that challenging; when you have to multiply that 100 times, 1000 times, that's when we get, you know, real challenges in the supply chain. And now, the fusion industry is really starting to think about that.
ML Aneeqa, let's come back in a second to the supply chain and the spin offs, because I think that's a really interesting topic. But what sort of materials are we talking about, that might be able to thread that needle between all of the different requirements that you've got there?
AK So, for the high heat kind of applications, tungsten is a really popular choice at the moment, which is being used for that divertor region. It could potentially be used higher up in the reactor as well. Currently, we use beryllium for some of our test tokamaks, but we might not use that in the future, because it's quite difficult to work with, it's very toxic, etc. So, I think there's a shift to try and use tungsten in those areas as well. People are also looking at novel alloys, because although tungsten is great - it's got the highest melting point of any metal, it doesn't erode that much, it’s quite strong - it's not perfect. It can still get damaged at those high temperatures. So, there's very varying degrees of research going into novel alloys, and things like that. So, one material I'm looking at the moment is CVD diamond, coated with tungsten actually, which again, very, very niche, low technology….
ML Sorry to interrupt. CVD diamond, for our audience who are not super experts, that's…
AK Chemical vapour deposition. Diamonds made in a lab.
ML Chemical vapour deposition. So, it's a lab diamond, but it doesn't look like a diamond. I mean, you're not trying to squish it all together and create something that you could wear on a finger. This is on a surface; you're depositing diamond onto a substrate of some sort?
AK Yeah, so it gets deposited on a substrate. We actually buy it from a company called Element Six. And it's just these kind of little square, tiny, tiny bricks, a few millimeters in size of diamond, that we're researching at the moment to see if we can potentially use that in fusion applications.
ML And what does that do? I mean, it sounds marvelous, to use diamonds, you know, tremendous. I'm sure it'll be the plot of maybe, what was the film about hydrogen, just recently. I can't remember, I’m blanking on the name. And now we could do another film on diamonds and fusion. But why would you use CVD diamond?
AK So basically, its thermal properties are the best out of any of the materials going. It can deal with these high heat loads, basically, that's the main reason. Again, it's not perfect. So, it could erode because it's a carbon-based material, but to try and counteract that, we're putting tungsten layers on top of it, to see what happens and to see if that helps it resist erosion and other damage from the fusion reaction.
ML So you're buying in these little teeny diamonds from a company that you mentioned, and then you're researching. Presumably you make a little sample of something and then you subject it to these terrible conditions, or as bad conditions as you can create currently in the equipment as it stands. So, it still is, it feels quite early stage. I mean, presumably there will be lots of spinouts, and companies that come out of this work eventually, or am I wrong?
AK Let's hope. It's very, very early stages. So, we're still just characterizing it for exposure to different kinds of conditions. So, we're seeing does it maintain those thermal properties when we deposit the tungsten on top, how is the adherence between the tungsten the diamond, because that can be quite challenging as well. So, for this specific, very niche project, it's very, very early stages. But there's quite a few other researchers doing other kind of low technology readiness level type of research in combination with people doing stuff much higher up, that's more established as well. And I think we need both, because in the near term, we need stuff that already works, and which we are already confident in manufacturing. But we need to always be looking at how we can improve and looking for other solutions as well.
ML Because we hear all about the actual fusion startups, you know, the Commonwealth fusion... the one in Cambridge, Massachusetts; we hear about the Spherical Tokamak in the UK; we hear about all of these companies. What we don't hear about so much is the way that there's this explosion of knowledge and potentially spinouts. We had, as I say, Seurat Technologies using the laser. But are we going to see lots of little materials companies? Or is it essentially going to end up being the big, I don't know, catalyst companies, the big metals processes that already exist, that monopolize this sector?
AK So, I think both. So, I think there is a shift to trying to get conventional engineering companies and manufacturing companies into fusion. So, I've definitely noticed that, people like Jacobs, or Stanmore or, you know, [inaudible], these kinds of big, big companies. And actually, so I'm on the board for the Fusion Centre for Doctoral Training, which is a training program for PhD students. And actually, last year, for the first time, we put on a fusion industry school, which is basically providing training for people in existing industries, who are now starting to get those fusion projects, so that they can see the more specific applications within the fusion industry. And that's something that's never happened before, so that's really exciting. But then on the other hand, you have smaller companies, as well. So, there's a company called Kyoto Fusioneering, which... they're not building a reactor, like Commonwealth Fusion Systems are, or Tokomak Energy. But they're building components for the reactor, and developing ways to test materials. So, they're kind of running in parallel, or trying to aid the delivery of fusion, while not actually competing with any of the other kinds of companies as well. So, it's a bit of both, I would say.
ML It must be quite challenging for the big companies. I come from an entrepreneurial background, and I know that large companies, they can't spend a decade, 15, 20 years producing these tiny amounts of - essentially - samples for people like yourself to go and experiment with.
AK I think that's definitely been a challenge. For sure, big companies don't want to produce small amounts, it's not worth their time. But I think as the industry is starting to grow, and they're getting more and more projects, where they're involved in larger scale things, they're definitely starting to scale up their involvement in the industry. And the hope is that in the next few decades, they will be the ones leading the way, not researchers; it will be an industry led endeavour, I believe, as we go forward.
ML How much interaction is there with other industries or other sort of technical challenges? You mentioned the Rolls Royce Trent, as being a high temperature environment, high stresses, toxic gases, all sorts of interesting stuff going on. Are you learning things - you and your colleagues - that can then be applied into... whether it's jet aircraft or whether it's other high temperature chemical processes? Is there some cross learning going on? Or is this just so specialized, that there's a kind of a clique of people like yourself that are just forging ahead, but on your own?
AK No, absolutely. There's so much crossover, even from very small things that you might think are insignificant. So, the fuzz that I mentioned previously, is now leading into research on how that could potentially be used in photovoltaics. So, in the solar industry, for example, which is so interesting. Anything with high temperatures has so much overlap with aerospace, or other kind of high heat applications and things like that. So, there's definitely a lot of crossover there. If we look at robotics, that's another area; anywhere where you need remote handling, or places that are very difficult to get to, there's been huge advances in robotics in fusion, and I think that's definitely transferred. Same with the superconductors that I've mentioned previously, the high temperature superconductors. Digital engineering is another big one. So, a lot of research to try and figure out designs for reactors, or novel materials, and we don't have the resources to do all of that experimentally, or we can't do some of the experiments that we need to. So, digital engineering is taking a big role in fusion and again, very transferable to other industries as well.
ML Let's move on if we could just to some of the engineering challenges, understanding that you are a materials science specialist. But I still think for our audience, I'm sure you'll be able to enlighten us. You've got some real big challenges to get enough energy into the material that you're trying to get to the point of fusion. And then you've got to get energy out of that, and somehow, ideally into the electrical system, although there may be some other uses for it. Where are we on the... Is there a parallel effort on engineering? Or is it more, well, hang on a second, we're still 1% of the way towards actually producing enough heat, so that it's not even worry about that?
AK So it's early stages, but it is happening, people are doing research into the infrastructure we need to extract the heat or the energy out, whether it's for electricity or other applications as well. So, the company I mentioned previously, Kyoto Fusioneering, they're looking into that, they're trying to demonstrate how we can... what infrastructure we would need, as well as academic efforts as well into researching the area.
ML Can you describe... Certainly, I would have thought you could do for a tokomak, but perhaps for some of these other architectures: is this going to be pulses of heat? And if so, are we talking one a second? Are we talking continuous? Are we talking 40... a second? What will this actually, potentially, end up being?
AK So at the moment, tokomaks run pulse, they don't run continuously. I think ITER aims to do pulses of 400 seconds, so I think that's the longest one. But that's not a prototype reactor, that's purely experimental.
ML Okay, but it is 400 seconds. From ignition, so then it's going to get hotter and hotter for 400 seconds? Or it's going to run for 400 seconds and then...?
AK Turn off. I believe that's the case; I might be wrong. Stellarator can run much longer, so I think they can run for... I don't know what's the longest it's ever run, W7X, but definitely minutes and minutes, maybe 30 minutes or so, maybe even longer.
ML Aneeqa, is the goal to run continuously, then? I mean, are we talking about... You know, I remember when I was learning to ride a bicycle, and if I could do one minute that was good, but ultimately, you want to be able to ride a bicycle. Is it like that? Or will it always be these pulses?
AK I mean, people are trying to see how we can run it for longer and longer. There may be technologies that develop for it to run longer; stellarators already can, inherently by their design. I don't think it's a problem if it does run in pulses, as long as we can store the energy that's produced in some way. So, whether it's with molten salt or another kind of energy storage facility. So, it may be that it runs, it generates this heat, it's stored with molten salt, and then you can use it kind of continuously. But at the moment, yeah, tokomaks, they do run in pulses and stellarators, they run a lot longer,
ML Presumably, the inertial confinement, and the magnetic, that would definitely need to be pulses, and the non-ignition ones, these would be producing, storing up energy, using it to create ignition, or non-ignition, and then getting a big pulse out, and then having to store or buffer the energy in some way?
AK Maybe I'm not so sure about the other technologies. But yeah, but for tokamaks, there would definitely be kind of pulses, at the moment. Again, that's not to say that the technology doesn't develop for them to run for much longer. But yeah, I don't know too much about the other techniques in terms of how they would operate.
ML There's a subtext here, which is, how far are these things from the market, from actually becoming commercial. And I look at it with knowledge of - whether it's turbines or heat pumps, or fuel cells, or wind turbines, or solar - all of the different things you need between the lab and actually starting to feed electricity reliably into a grid at scale. It does feel still quite far from commercialization...
AK Yes, I definitely think it's a long-term solution, we're not going to have fusion electricity on the grid tomorrow. I think we will in the second half of this century, so after 2050 or so, I think we will be able to have fusion electricity on the grid, but definitely a longer-term solution, not a short term one. In the short term, we need to be using traditional nuclear and some of the other renewables that you've mentioned, whether it's wind, whether it's solar. We need to be throwing everything we can basically at the climate catastrophe.
ML I wrote a piece in response to Vinod Khosla, in The Economist By Invitation. There's a digital column, and he said, we shouldn't be doing wind and solar, because they're intermittent and they don't do this thing called baseload. And we should... we've got these tremendous opportunities and very promising technologies, and one of the ones, of course, that he pulled out was fusion. And my response was that I can't see it producing... if you look at wind and solar, that are now 15% of electricity globally. How long would it take for fusion, and some of the other technologies that he mentioned, to get there? It felt to me, and you've just said it yourself, it feels like a sort of second half of the century thing. But you've got these startups, who are all promising, presumably promising their investors as well, not just the public, that they're going to be able to produce a replicatable design before the end of this decade, and start feeding into the grid, presumably, in the 2030s.
AK I can't speak for any of the companies. I will be very happy if any of them do this, right? As you mentioned before, it's a bit of a Wild West, there's lots of people trying lots of different things. And I would absolutely love if one of them is successful, and can do something sooner rather than later. So, in science, we can never say never, but just from my kind of broad overview of the field, and just using information that I'm aware of, obviously, I don't know the ins and outs of all of these companies, and a lot of their stuff is kind of very, hidden within the company, it's not available publicly. My feeling is it is more long term, and we should be using established technology in the near term. But that's not to say that some of them won't do something sooner, and I'd be very happy if they do.
ML If you look at the data development of... Presumably you're more than familiar with JET, more than familiar with the Spherical Tokamak with ITER? How far are they from this kind of 100x, or, you know, 100x improvement, just to get to breakeven. So, presumably, we're talking about really 1,000x improvement is what's needed? What's your sense of the timing on that?
AK So, you know, we talk about JET and ITER; they were never designed to be reactors, they are purely experiments. So, they're not designed to generate electricity or put anything on the grid, so that was never their purpose. It's more to test the technology and, the fundamental science. But in terms of having a fusion reactor, similar to one of those, I think the same, it would be the later half of the century, before we could have them actually contributing to our net energy baseline.
ML There'll be some people out there disappointed. Also, I know there'll be people out there saying, oh, but we've got, we've invested in this other startup that's not a tokamak, and we're going to get there much, much sooner. And, and I suppose you'll say, well, you know, but I can't comment on that, because that's not my area of particular specialization.
AK Yeah, I won't be able to Michael, as you've correctly... Yeah, I don't know their technology, so I can't comment on them. But like I said, I think all the fusion community are really working together and collaborating a lot. And I think everyone will be very happy if someone is able to do it quicker. And I think you know, there are so many different designs. And I think as a community, we shouldn't be scared of failure, either as well. It's like any big race towards something, there'll be some that do it, and some that don't. And as long as someone does it, I think none of us really care.
ML I'm definitely in the camp that says we should be investing in this because, first of all, there will be plenty of demand for clean energy in the second half of the century. I mean who knows, we may need to be doing large scale, direct air capture of carbon to try and remediate the awful things we've done. So, there's not going to be a shortage of demand, even second half of the century. And the spin offs; I'm also very convinced that the spin offs, that knowledge and learning and all the things that we'll get out of it, even if we never manage to get a fusion reactor itself - or not for many decades - those will still be worth it. But I do push back against those who think it's going to be easy or quick, or that it is the solution, therefore, we shouldn't be doing what we've got now, because we might have some tremendous breakthrough. And it feels to me like, if we're still doing this sort of experimentation, we may know how to do it by the end of this decade; and then we may have done it a few times by the end of a decade after; and then we may do it, you know, 500 times or something like that, that's meaningful in scale in about... I mean, the old joke is that it's three decades away, isn't it, and it always will be.
AK Yeah, no, absolutely. No, I totally agree. I don't think any technology is a silver bullet solution. Everyone has their own diverse needs, depending on their geography, their energy requirements, the state of their grid, so there's no one energy source that is going to be amazing for everyone and going to fix all of our climate worries. There's always a loser, whatever technology you use, and we just have to kind of make a balanced decision and approach to things. But I think having a really diverse source of clean energy is really, really important for everyone globally, whether it's wind, whether it's renewables, whether it's nuclear fission, and then hopefully, in the long term, fusion. But I think having both a short term and long term strategy is very important, because I think the world has suffered from people just thinking in the short term and trying to look for easy fixes and quick solutions. But real progress requires a lot of effort and long term planning and work. And I think, yeah, we do need to look at the long term.
ML I should also say that some of the companies, even to me, as a non-specialist, do look more promising on the engineering front than the tokomak approach, I've got to be honest. So, Helion, which is a portfolio company of Capricorn Investment Group, who support this show - but not because of that - I like what they're doing. They accelerate... they create a plasma and accelerate it together, but they're not, they would not be extracting the energy using heat, it would actually be because they've got such a high beta, the magnetic field itself that accelerates, as this plasma generates energy, it pushes back and potentially generates electricity directly. So, the same coils that accelerate it together could then extract energy. It kind of feels very elegant, but I haven't got a strong sense of the engineering challenges that they will have with that approach.
AK Yeah, for me, I also am not an expert in this field, I just know what you've told me and whatever they've put online, so I don't know the fundamental technology and the details that they're working with. But I can imagine, engineering-wise, it's going to have its own challenges associated with their approach, as well. But there's definitely such a wide variety of approaches, it's difficult to keep on top of all of them, for sure.
ML In 2021, there was something like $6 billion of capital that poured into fusion startups, and there you are in your office in Manchester. What do you think? What do you feel when you see those flows of money? Are you happy? Or is there a part of you that says, this is going to end badly, that a lot of this money is going to be wasted?
AK No, I think it's very exciting. From starting when it was purely lab-based, and there wasn't any of this kind of investment and stuff, it was still really interesting to me. But I think having it enter the public conversation, and people being more aware of it and creating more opportunities for people, I think this is really, really cool and exciting; and bringing people from other industries to work into it, as well. Because we've about the material challenge, but I think our biggest challenge is people: having enough people, it is expanding at such a rapid rate, and we've got so much work to do, having people with the relevant skills, being trained from an early age or learning about it from even primary school level, or from people in existing industries being trained and brought in, there's so much growth we need as an industry in terms of skilled people. So, I think that's also a huge challenge that we're dealing with.
ML I hear you there, and I absolutely agree; to have some really exciting engineering challenge that gets kids into physics, that gets them then going off to study STEM, undergraduate, graduate, do doctorates, postdoc, etc. I mean, it's absolutely essential. Now, you talked about one initiative along those lines that was encouraging. What exactly was it doing? Where was it based?
AK The Fusion Industry School. Ok, so that's not targeting young people, that's for people already in industry, and that was run through the Fusion Centre for Doctoral Training. So, we had one week in York and one week down in Oxford, working with UKAEA as well, so it's split across the two sites. I'm also on the Fusion Centre for Doctoral Training board. So, we train PhD students entering fusion in a whole variety of different kinds of areas, in the UK. So, it's a collaboration between York, who's the lead University, Manchester, Oxford, Durham and Liverpool. So, that's a really wide range of universities all working together.
ML I was astonished to see, there's a map out there, with 36 different tokomaks around the world. There's tokomaks in Brazil, in North Africa, in all sorts of places in Asia; far more than I would have thought.
AK Yeah, it could be even more than 36 I think, but there's definitely been hundreds of tokomaks built around the world, so it's a really big area of research. Even ITER which is being built in the south of France, is an international collaboration between seven member states including the EU, US, Korea, Japan, China, India and Russia. I think that's seven, I may have missed, some of them off, but a huge global effort.
ML And one issue that, if you don't mind, I can't leave the conversation without raising the topic. Gender equality in science is such a big challenge. What does it look like in fusion? Are you an exception, or is it 50/50? How are you doing in fusion?
AK So I would say it's, across all sciences, it's not great, I have to say. And I don't think fusion is any different from that, unfortunately. I think it is changing, especially at the younger level, we are seeing more girls getting into STEM subjects. Because there are a lot more initiatives these days, especially targeting younger kids in primary school and things like that. It does vary internationally as well. So, some countries, they do really well, and they have more women than guys, and some have - well, the majority - more guys than girls as well. But I think another thing to consider is the retention. So, it may be that we're getting quite a lot of women in, but as you get higher and higher up, there are fewer women in those positions of power, if you will. It is changing, but I think we need more targeted initiatives, more specialists working... You know, I'm trained as an engineer, I'm not trained in gender studies, or finding the solutions for these things, I can only talk from my own personal experience. But I do think having more mentorship schemes and more targeted approaches that do target women, and all other minorities as well; it's not just a male / female issue, whether we're talking about race, disability, neurodiversity; we need to have like diverse groups of people to solve these really difficult challenges, because everyone has a different viewpoint, and getting all those voices together is really important. So, a work in progress, but I hope it's changing for the better.
ML Indeed. It's an issue that I've been tracking in energy more broadly, and we've definitely seen a shift. From the early days, when I started New Energy Finance, other people were having conferences which had 7% women, and I put my foot down, and we got to 30% reasonably quickly. And I think now - I'm no longer New Energy Finance, Bloomberg New Energy Finance, but I think it's now around 40%. But it's taken explicit work, and I don't think that the initiatives in those other areas in race are... Actually, interesting: physics is probably better on neurodiversity than a lot of topics. There's at least from my experience, there seems to be a broader range of characteristics. But on race, I think it's probably still very poor, even in this country, and so it's something that needs work. I don't know whether I can be of any use. But certainly, I would encourage and I endorse entirely the points that you've made on that.
AK No, I think any support is always appreciated. And, yeah, I think it just comes down to making sure that everyone has access to the same opportunities, which is something I'm really passionate about.
ML Very good. Well, thank you so, so much for coming on Cleaning Up sharing some time and some insight with us here today. I wish you luck... may your... may your decades become just years... I'm not quite sure what sort of blessing to give to this incredible work on fusion and materials.
AK Let's hope Michael. You know, when I first went into fusion, someone would ask me, when is fusion going to happen, I said I'd have some gray hairs, but unfortunately, I've already got some, so I hope it comes sooner rather than later.
ML By the way, if it's any help, when I started to cover the renewables industry, the big question was, when will it be grid competitive? In other words, when could renewable electricity be competitive at the wholesale price level with other forms of electricity? And it was always asked by people who fundamentally thought the answer would be never. And the answer was 2015. So, I started 2004, it took 11 years, and it was grid competitive. And it's now the cheapest form of electricity that there is. So, who knows? These things can sometimes go much faster than we think.
AK Yeah, absolutely. And yeah, it's good to have those positive words to end with.
ML Very good, thank you very much. Bye bye.
AK Thank you, Michael.