Ep122: Sir Chris Llewellyn-Smith "Solving for Storage"
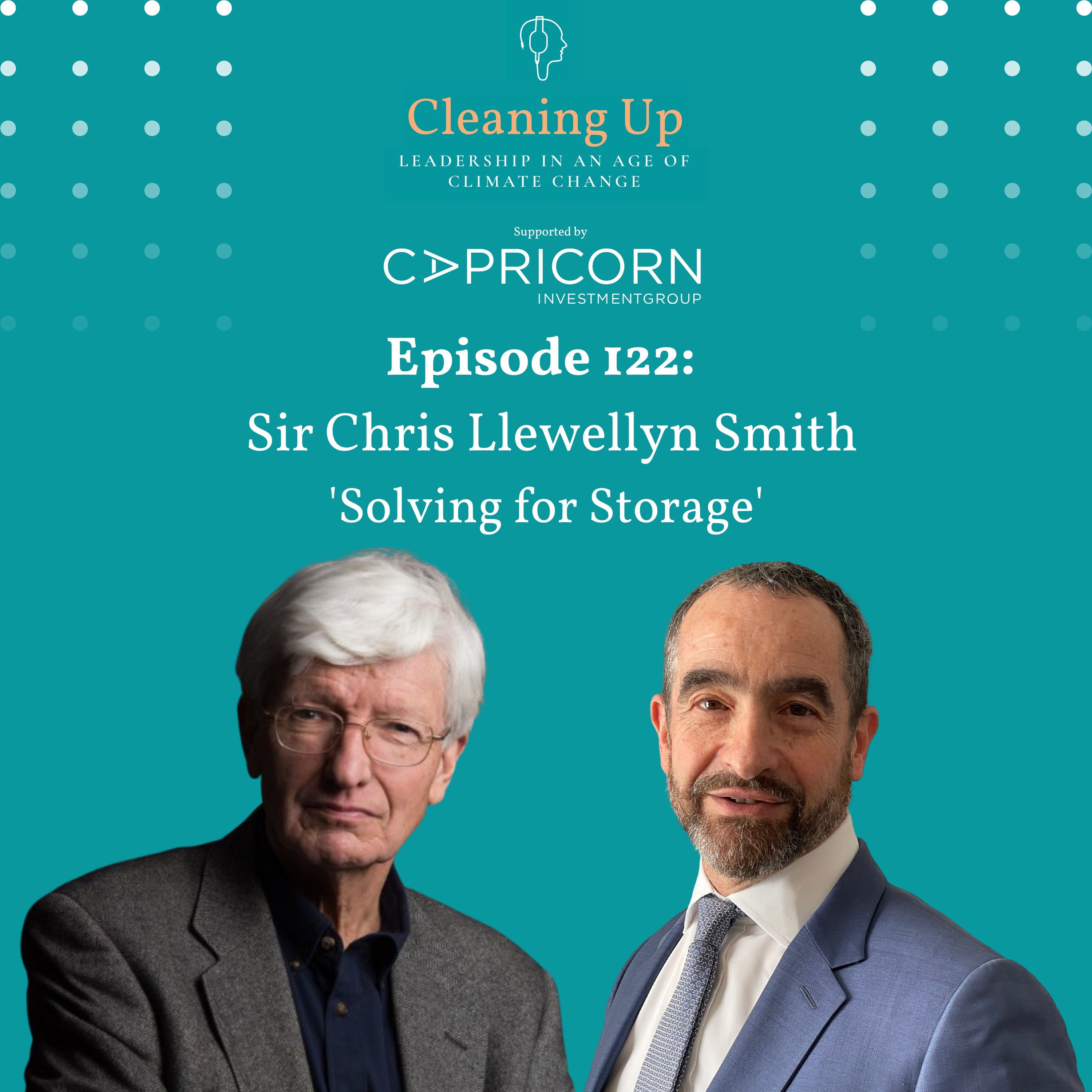
This time on Cleaning Up, Michael hosts Professor Sir Chris Llewellyn Smith, one of the UK's greatest living physicists, for a fascinating and detailed dive into the UK's energy storage requirements as it moves towards a fully carbon-free grid.
Sir Chris is Emeritus Professor of Physics at the University of Oxford, and a former Director-General of CERN. At CERN he steered the funding and commissioning of its Large Hadron Collider, and as a theoretical particle physicist, Sir Chris predicted the existence of the very particle - the Higgs Boson - that the Large Hadron Collider went on to help discover. Sir Chris is likely the only person ever to have been knighted for "services to particle physics."
In the last few years Sir Chris has been busy leading a forthcoming Royal Society study on large-scale energy storage as part of the net-zero transition. He and Michael discuss the significance of storage to a newly configured, renewable grid, the likely cost of stored electricity, and the role of salt caverns in hosting hydrogen stores.
Short on time? Read the Edited Highlights here: CLICK HERE
Relevant Guest & Topic Links
Sir Chris discussed the energy storage requirements of a net-zero UK at a virtual conference in March 2020. His slides are available here: CLICK HERE
Discover more about Chris’ storied career here: CLICK HERE
Watch Episode 104 with Yanis Varoufakis here: CLICK HERE
Watch Episode 115 with Jorgo Chatzimarkakis here: CLICK HERE
Watched Episode 116 with Tom Samson here: CLICK HERE
Guest Bio
Sir Chris Llewellyn Smith FRS is a quantum field theorist and Emeritus Professor of Physics at the University of Oxford. Chris was Director of Energy Research at Oxford from 2011 to 2017, Director of the UK's fusion programme (2003-2009), Provost and President of University College London (1998-2001), Director General of CERN (1994-1998) when the Large Hadron Collider was approved and construction started, and was the first Chairman of Oxford Physics (1987-1992).
Chris holds a BA in Physics with First Class Honours from the University of Oxford, as well as a D.Phil in Theoretical Physics. He holds honorary doctorates from universities in the UK, Spain, Canada and China. He was knighted in 2001 for “services to particle physics.” He is a Fellow of the Royal Society and was awarded their Royal Medal in 2015. Chris captained Oxford’s cross-country running team as an undergraduate in 1963. After completing his doctorate in 1967, Chris worked briefly in the Lebedev Physical Institute of the Russian Academy of Sciences in Moscow.
Michael Liebreich Chris, thank you so much for joining us today on Cleaning Up. Can we start, as I always do, by asking you to give a thumbnail bio, in your own words - and you have such an illustrious career that it'll need to be the short version - but tell us who you are, in the thumbnail version.
Chris Llewellyn-Smith Okay, so by background, I'm a theoretical particle physicist. In the 60s, 70s, 80s, I had a hand in constructing what's called the Standard Model of particle physics, the idea that protons and neutrons are made of quarks and gluons. And also, I was able to show that we had to have something like the Higgs Boson - that attracted some attention. And then, for complicated reasons, although I'm a theorist, I found myself in the 90s as Director General of CERN in Geneva. I'd been working a lot on the physics case for building the LHC, but then I found myself as Director General putting together the proposal, with all the money that was needed, persuading the governments, bringing the Americans, Japanese, in. And I got the project funded and construction started, and the rest is history. Then I left, and since then I've done a number of things, I worked in fusion for a time. But for the last 15 years, I've been working primarily on more or less all aspects of energy supply and demand. And the last three or four years, I've been leading a major Royal Society study of large scale electricity storage.
ML We're going to focus on that study for the Royal Society today. Although before we do that, two things. One is, you talk about the LHC. That's Large Hadron Collider, which is ITER in Cadarache, in France, correct?
CL-S That's the Large Hadron Collider. I was involved with ITER - that's a completely different thing - in my career in fusion. I was the chairman of the board, as a matter of fact.
ML Okay, I've commingled two things. But the other thing I wanted to just touch on, because I absolutely love the wording, is in 2001, you were given a knighthood, and it was for "services to particle physics." And I don't know that anybody else can say that...
CL-S I think that's true, but I'm not certain.
ML Very good. Well, we've established you're bona fide as a scientist, and now we're going to move on to that Royal Society study. And that was, you've described it in talking to me, as this sort of system study, and we talked about resilience, but you've also described it as a storage study. Can you start by talking about the terms of reference of that whole piece of work?
CL-S Well, we didn't have any formal terms of reference. But what we decided to do at the beginning was look at large scale electricity storage. So, an underlying assumption of all this, which I think everybody would agree to, is that as we go to 2050 - and we're looking ahead to 2050, thinking of the end point, but haven't worried about the path very much - we think by then a very large part of our electricity will come from wind and solar, because they're cheap. But of course, the sun doesn't shine the whole time, the wind doesn't blow, they vary a lot. So, if you have a lot of wind and solar, they'll always be times when there's not enough. So, you've got to complement it with something else. So, there's basically two things you can do. There are times when there's too much wind and solar; so, you can store the excess, and use it when there's a deficit. And - or - you could have a mixture; you can find other flexible sources of supply. So, we've studied the storage option. And then, we did compare with one of the other options. We think storage is the cheapest option.
ML You're into the land of trade-offs, aren't you? Because you can have over-capacity on generation, and then that enables you to have less storage. Or, you can have no over-capacity, and then store everything extra that gets made at any time when it's very windy and sunny, right?
CL-S That's right. So, there is a trade-off, and there's a minimum in the cost. You can't get everything from wind and solar, however much... I mean, there are periods, maybe even weeks occasionally, when there's no wind and solar. So, we find that somewhere between about 25% more wind and solar being generated than electricity demand, and something like 60%, 70%, there is a sort of flat minimum in the cost; you need less and less storage as the wind and solar supply goes up, but you're paying more and more for the wind and solar. So, one's cost is going down, the other's going up, there's a large flat minimum.
CL-S One of the takeaways, I think, from your work, is that you don't actually have to get it exactly right, because it's quite a flat plateau, that optimization: as long as you are somewhere in that zone, between something like 25% over capacity relative to demand, and 60% or 70%, it's fairly flat, because you're adding capacity and removing storage, or vice versa.
CL-S Well, that depends on the storage technologies you're using [that] these numbers actually detail. But in the case that we looked at first, which we'll come to in a minute, the ideal number is about 33%; 20% might be a bit low. But it's quite broad.
ML And before we dive into the detail of that case, let's probe a little bit more some of the boundary conditions, or what you've got in there. Because that is... we've dived into this. I think what we do agree - I think pretty much everybody would agree - lots of wind and solar. But not everybody would agree that it's only wind and solar. And so, we've got nuclear, we've got biomass, we've got, you know, a number of other - biogas - and of course, interconnections with our esteemed European partners, which we currently already have something like eight gigawatts of interconnections. So, what have you assumed on all of those dimensions?
CL-S So, what we've done in the first instance, we've neglected them. So, we've said, let's look at a very simple case, as a benchmark: could you do it without those things? What would it cost? And then we ask, what happens if you add them? But it gets very complicated when you start adding many things. But they are not.... I mean, there will be some nuclear; presumably, Hinkley Point in the UK will get built, so that will be operating in 2050, if it's ever built. There will be some burning of waste, and some biomass, which there is at the moment, there'll be a little bit of hydro. But they're not huge, they're not going to be huge on the scale of demand we're going to have in 2050. So, in the first instance, we neglect them. As far as interconnectors are concerned, we've taken the view that... The difficulty we face is that they you get wind droughts. You get periods of a couple of weeks, every 10 years or so, or even longer periods, where there's almost no wind. And if that's true in the UK, it's going to be true across Northern Europe. So, we think it will be not prudent to build a system which couldn't work if we couldn't import. So, we haven't concluded interconnectors. On the other hand, they will be there, and they will add a degree of flexibility. So, they'll help manage the system.
ML If I'm right then, what you've said is, you're doing some sort of an upper bound, based on the worst case, which is: when we need the power, Europe needs it too, and therefore interconnectors can't be relied on.
CL-S That's right. So, in some sense, throughout, we're looking at the worst case. So, for example, we look to begin with at one mode of storage, which we cost very, very carefully. And then if you say to me, hey, but adding something else might make it cheaper. If it made it more expensive, you wouldn't do it. If it makes it cheaper, you would. So, by looking at one system, you're getting the worst case, if you like.
ML And what about my favourite - and I say that because I'm an investor - Xlinks, where you've got wind and solar in North Africa, not coming through the European grid, but right around the outside, subsea, and into the system. So, it's no different in a sense from offshore wind, except that it happens to be onshore in Morocco.
CL-S Okay? Well, I have looked at that. But it's not able by 2050, when our electricity demand will be twice what it is today, it's not going to be able to - it'll be very helpful - but it's not going to be able to provide a major fraction. So again, in the spirit of saying, let's look at the worst case, we haven't taken it into account. We also worried about systems that might be politically vulnerable. Now, at the moment, North Africa is fairly stable, but I don't think we can be sure that that will be the case in 2050.
ML The reason I'm probing here is that actually, just at the end of last week, the Climate Change Committee produced an analysis of a decarbonized electricity system by 2035, which is a commitment that the government has also made, and how we might do that. And what they have come up with is 70% wind and solar, and then 20% is nuclear and other dispatchable forms; so nuclear, biomass. And then 10% is this flexible... I think 8% is flexible coming out of storage, and a couple of percent, they say, will still be unabated gas.
CL-S I haven't read their report, so I tread in this area with caution, but I find that quite difficult to believe. Because what we find is, if we add nuclear, for example: first of all, you don't want to operate nuclear flexibly; once you've built it, you want to operate it. If you add nuclear, it will put up the average cost of power we get with just wind, solar and storage, unless it's cheaper than the average that we get. And by all accounts, it's not going to be. And the reason for that is very simple: if you add nuclear, you've effectively removed part of demand, just forget about it. So, the thing that you're left to deal with - which is the difference between wind and solar supply and demand - has become more variable. So, the cost of meeting that from storage goes up. So, if you add nuclear to a system with wind, solar and storage, the bit coming from wind and solar storage becomes more expensive. So, you shouldn't add it unless it's cheaper than what you started with. And it's probably not going to be.
ML Unless you can use it in a way which is flexible. But before you answer that, Chris, let's come back to nuclear. Because otherwise we'll get the nuclear tail wagging the dog of the system that you have designed. And we had a wonderful episode a few weeks ago with Tom Samson, who's the chap leading Rolls Royce SMR, talking about how much it could cost, and how flexible or otherwise. And we explored a few ways that you might be able to use nuclear, that are flexible, that actually don't cause the problems that you've described. But let's get back to the model that you've run, because it's got such powerful learnings... And as you say, then you can always flex it, and see if you can improve on that. But there are some core outputs, aren't there?
CL-S Yes. So, if we go back to that: first of all, as I said, to discover what you need in the way of flexible generation, or flexible supply from storage, you have to look at demand hour by hour. So, we have a model of what we think, not just what the level of demand will be, but how it will vary from hour to hour through the year; and you have to have a model of wind and solar supply. So, the model of wind and solar supply we have was based on real wind and solar data turned into artificial energy, as if we'd had all this generation going back over 37 years. And at first I thought, oh, that's quite good, 37 years, I don't know anybody who has looked at anything like that. And then various things we were finding made me worry that that's not enough, because there are these very long term variations. And it probably isn't enough. So, we add some contingency. We can come back to whether it's enough - it doesn't cost very much, as a matter of fact. But one of the major messages from this study is that a lot of people have looked at storage and said, oh, we'll look at a couple of typical years, maybe a bad year. That's not enough: you will get the wrong answer by a very, very large factor. I mean, we really find there is a need to store energy for 10 years, things like that, in some cases. So, looking at a few years; don't take seriously anybody who has modeled storage who hasn't looked at a very long period.
ML And I think that's another key learning. The first one was that we don't have to get this exactly right, but we definitely need contingency; we need more generation than 100% of annual demand. But what you're saying is, we might need quite a bit more, because you can have bad wind years, you can have them one after the other, and you need a lot of data - 37 years is not enough. So, you can't go back further than that, you're going to add a contingent on top of it.
CL-S Yes. So, actually, you could go back, but nobody's done it. The wind and solar [inaudible] exist, but they haven't been turned into artificial electricity generation; it's an exercise somebody should carry out. So, we've added to the store - it's just a finger in the air - we said, let's make the store 20% bigger than we thought we needed. Now, the interesting thing is, that only adds £1 a megawatt hour to the cost. So, that's easy; if you say, we want 40%, I can do it; couldn't do 1000%. But the point about that is that - on the basis of what the what we've looked at, 20% seemed my best guess - but the contingency would be built last. I mean, if we're going to build a lot of storage, we're going to build what we need, and then we'll also add a bit. So, by then we'll know better what we're doing.
ML Let's understand that, because adding 20% more storage and having only £1... And for those who are not totally fluent in electricity prices, we're talking about generating wind should be something like £37 per megawatt hour, that's the most recent round of bids. And generating natural gas might be £40, £50 power. So, adding one pound doesn't sound much, but why is that? Can you describe... Give us the shape of the system. How much wind? How much solar? Where is it? How are we doing this?
CL-S Okay, so, in a minute, I'll have to go back to what storage we're assuming, which we've not discussed yet. But it's very simple: in the system that we're looking at, with nothing but wind, solar and storage, storage at the energies that minimize the cost are only providing something like 15% of the power. Now, the storage cost is broken into three parts: the part of converting the electricity into a storable form; the cost of storing it; and the cost of converting it back into power. And they contribute equal amounts, roughly speaking. So, the cost of storage only contributes 5% to the average cost of power. So, you can add 20% of it, and it doesn't have a big effect.
ML And this is the because, first of all, you've got 85% which is just about the costs of wind and solar, and they're getting cheap. And then you've got 15%, but even that, adding more storage... And we are talking here, and I'm going to lead you onto it, we're talking about hydrogen in salt caverns, is your sort of base case. And just having more salt caverns only affects that middle third of the cost of the storage, right? So, that's why when you multiply the percentages, it's actually not terrible just to have too much storage.
CL-S That's right, it's okay.
ML Now, you got down to hydrogen and caverns by a process of... was it intuitive elimination? Or was it sort of analytical in elimination? And what did you eliminate?
CL-S It was what we expected, but it was analytical elimination. So, when you look at these very long term variations in wind supply, which are to do with what's called the North Atlantic Oscillation changes. And by the way, the danger of them is, the periods when there's very little wind are correlated with periods when it's cold, so there's special demand for electricity. We haven't treated the correlations as carefully as I would have liked, but they are a sort of second order feature. So, when you look at this, you discover you've got to have some storage, which will last ten years or something like that. Now, that means that the cost per unit volume of storage has got to be very low. I mean, you'd be bankrupt immediately if you tried to use batteries. Batteries, if you use them to store, you've got to fill them up and down very, very quickly to get your money back. But if there's a large capital cost sitting there, just storing things, it better be not a large capital cost! It better be as small as you can get it. So, in fact, for long term storage, there are really only three options - for very long term storage - though we're going to add some others. And those are making hydrogen; making ammonia, but that's more expensive because ammonia is made from hydrogen. And not only that, making it takes energy, so it's going to be more expensive. And the other thing is to make hydrocarbons artificially, those are called synthetic fuels. If you make synthetic fuels, you start by making hydrogen, so you might as well store the hydrogen. So, for the long-term storage, it's very simple: we looked at hydrogen. And then - we can come back to this later - we asked, maybe there are some shorter-term storages which would lower the cost, and there are.
ML Okay, let's come back to those. Because, for the audience, there'll be some out there who have not spent a lot of time looking at storage, and for them, it's a battery. And you said, that doesn't work, right? And that's just because the cost is so high that you have to use it a lot to get your money's worth. But what about some others? Can we not do it from demand response? Can't we just shut down industry, or ask people to turn the thermostats down?
CL-S The problem with that is... It will help and it's built into the model of demand that we used, actually, that that's being done. But the difficulty is, you get these periods, successive periods of very low supply. Now, we can reduce our electricity demand for a few hours; we could reduce it for a few days. But we're talking about having to lose half our electricity supply for weeks. Now, if the government wants to lose the next election, that's what it makes sure happens.
ML And it could be a few weeks, and then it could get better, but then get worse again for another few weeks straight after, right?
CL-S That's the problem. They can be repetitive, and that's why we put contingency in.
ML So, that's demand response. Demand response, no. Batteries, no. What about pumped hydro? I mean, everybody knows that the people who have really got deep penetration of wind and solar have generally done it in countries that.... It's Costa Rica, it's Norway. Can't we do that?
CL-S Not in the UK. The pumped hydro... Well, I mean, if you looked at the northeast United States, for example, there's a lot of capacity for hydro in Ontario, pumped hydro can play a big role. But the present capacity of pumped hydro at the moment in the UK, if you filled it up, and then you drained it, the maximum you could drain it, you would get 30 gigawatt hours. Now, your audience may not like the units, but we are wanting 30 terawatt hours. That is to say, the amount of pumped hydro in the UK is too small by a factor of 1000. Now, you could say, build more; the capacity doesn't exist to build more than another 20%, 30% or something.
ML Okay, very good. My next contender would be flow batteries; everybody loves a good flow battery. So, it's like a fuel cell, but the electrolyte moves through it from one tank to another, the tanks can be as big as you like.
CL-S Yes, it's like a battery, but the electrolyte is moving. Flow batteries are very interesting, and they can play a role. But they're going to be too expensive to do the long-term stuff. They may help you in the intermediate term, but at the moment, they're very expensive. And the reason that they're expensive is they rely on vanadium, which is an expensive material. Now, there are people, including British companies, who are developing flow batteries that use different materials. So, watch this space; if those come on, that could play a role. But in the category of storage where you've got to turn over the contents in a couple of weeks or less to get your money back: now, there are a whole class of storage technologies there. Flow batteries is one, but today they're not really there. Compressed air - we'll come to that in a minute in more detail - is the one we studied actually. But then there are others. There are things called Carnot batteries. Now, this is stacking up a big pile of rocks, blowing hot air through them, they get hot, and then you get the heat back later to make power. Siemens were building one in Hamburg, they built one, and they said it's going to be followed by a bigger one, but they've abandoned it, for reasons a bit unclear actually. That's quite an interesting technology. But we think that of that category, the one that we've chosen as a sort of example about whole class of things - we can't study eight different technologies - we studied compressed air storage.
ML Before we do that, I just want to tick off my last contenders. And, I hate to say it, I don't want to call them no hopers, but... We had a bit of a controversy on Cleaning Up with Francesco Starace, who is the CEO of Enel, the big utility in Italy, who was a fan of gravitational mechanical storage. And I was very surprised, because in all other things, I pretty much agree with him entirely, but on that one, I couldn't see the logic. Have you ever looked at it in any detail?
CL-S Yes, we have looked at it. There's another one being done in Italy, that's quite interesting, which is compressed carbon dioxide storage, which has the advantage of... I didn't mention liquid air storage; that exists in the UK, actually. So, you liquefy air, and then later you let it expand and it turns a turbine. So, that, you can't get very big units, but a lot of small units could have a role. So, that's one of our contenders, compressed carbon dioxide also. The gravitational ones, there's a number of companies, and they can play a role in very short term storage, for which we are going to need batteries, by the way, or something else, like gravitational storage, to deal with outages. I mean, what happens is, you get suddenly, a power station goes down, a power line collapses, or the proverbial halftime cup of tea in the cup final, and there's a sudden surge in demand. So, to keep the system stable, you have to have some things that can respond in milliseconds. And hydrogen, and so on, can't do that. So, we're going to need batteries or something like that. And I think gravitational energy can do that. But when you look at the amount of energy they can store, they just can't hack it. I mean, think about it: I just told you that acres of water, letting that drop through a dam, can only produce you 1,000th of what we need. If you start [talking] about dropping weights artificially... I mean, you're not going to have acres of concrete sitting up there, 30 metres deep.
ML Right. I did the calculations for a one kilo weight, and in order to get the same energy out of dropping a one kilo weight, as you get from one kilo of hydrogen, you'd have to drop it from 13,000 kilometres. But of course, at which point, it's not dropping anywhere, it's off in orbit, doing whatever it's doing.
CL-S That's right. I mean, I think there's a British company, Gravitricity, and good luck to them. They want to put down old mine shafts and things like that. Could work.
ML But these are these are tiny, tiny, tiny, tiny amounts, compared to 30 terawatt hours.
CL-S Very rapid response storage, they can deliver. That takes very little energy. So, we cost it, we assume batteries, but we don't...
ML I'm assuming all of that goes to either demand response, batteries or some kind of synthetic inertia. And we should move back to the core of the study. Because you've thrown out this number of 30 terawatt hours, but you've also spoken to me about 80 terawatt hours, and 100 terawatt hours. So, under what conditions do we need what?
CL-S Okay. So, that's a difficult question, because it depends.... I'm always being asked, tell is what volume of storage we need. And it's not a very well defined question. First of all, if there's some nuclear, you're going to need less. So, you know, things like that. But also, as I said, it depends: there's this trade-off between how much you're prepared to build of wind, and how much storage. So, in the range we are looking at, we had to store... I don't know how 30 got in the conversation. Oh, because we were putting 1000 times hydro. But actually, it's more than that. We need, with just hydrogen, we need between about 60 and 100 terawatt hours. That's the heat capacity of the hydrogen. Now, to put that in context, the UK today is using about 300 terawatt hours a year of electricity. So, the energy that's got to be the capacity, has to be about a third of the annual electricity generation in this country, something like that, depending on what units you use and so on and so forth.
ML And one thing we haven't touched on, and we need to, is that demand is going to go up, right? We didn't talk about the demand side; I'm assuming - I know, because I've seen some of the output already - that that is going to approximately double, more than double in that period up to 2050? And that's because of transportation and heating going electric, I'm assuming?
CL-S Yes. I mean, everybody agrees it's going to go up a lot. How much is not clear. And that depends on how much you think heating will be electrified. There are people - I'm not one of them - who think a lot of our houses will be heated with hydrogen, I'm not on that bandwagon, and so on. And it depends how we're going to deal with industry, if we're going to electrify, or burn hydrogen to make the high temperature heat, or whatever. So, you can get a range of predictions. So, we took one; we need an hour by hour model, and a AFRY consultancy very kindly, who built a very careful model, lent me their model, because making every hour of the year is not so easy. And that has 570 terawatt hours, so that's roughly twice the demand today for electricity. But then I said, we can't just publish with that, because somebody will come along and say, how do you know it's not all different if you've got the demand wrong? So, we looked at two other levels. So, we looked at 440, and 700, so plus or minus 130. So, those span the range of models you can get there. Now for those other ranges, we didn't have a careful model. So, we took AFRY's model, and we did violence to it - not with their approval. And we moved a lot more heating to be electrical, for example, than the upper model, and the lower one, we had less electrification of other things, and so on. And when we did that, there's almost no change in the cost of power. In the upper case, you need more wind and solar, and you need more storage, but the cost, it just scales up and down. The cost of the electricity you get out of the system is roughly the same. Well, within 1% the same, actually.
ML So, that brings us to the $64,000 question; it won't be $64,000, but... What is the cost of power, then, per megawatt hour? And you've got an average for the system. You've got the amount for the power that comes straight out of wind and solar to a consumer, and you've got an amount for the bit that goes through power storage. And then those two last ones have to average to the total system cost. So, where are you on that?
CL-S Right. So, if I look at the case that I was mentioning, that we have wind and solar is 33% bigger than demand. If I assume that wind and solar costs £35 per megawatt hour - I'll come to different values in a minute - then I've got to pay 1.33 x £35 for the wind and solar. So, that gets me to £48 or something like that. Then there's 15% that at that level has to come from storage. Now, the storage that will deliver 15% costs me about it, in our middle case, £80 per megawatt hour. So, I've got 1.33 times £35 plus 15% of £80, which is £12 - I can do that one in my head. So, when I put those together, I end up with a cost of about £60 a megawatt hour. And then I say, I've got to add a bit of contingency, I've got to have - this is for the electricity fed into the grid, by the way, because I didn't want to get into the increase of transmission costs, because that's what the model we had from AFRY... So, I add about £4 - £1 for providing batteries for this short-term fluctuation, £3 for transmission. So, in that simple model, I end up at £64 per megawatt hour. But that's a central value. We then say, hey, but wait a moment, we had to assume a discount rate. And then we had to assume, we had different costs of storage. So, we varied the cost of storage by plus or minus 50%. We said this is what we think it'll cost; that's what all the experts - or most of them - think, but we don't know. Then we said maybe wind costs £30, maybe it'll cost £45. And then we also said maybe the discount's 10%. So, we got a range, including the contingency and the extra transmission, from about £52 - that's with 5% discount rate, £30 a megawatt hour for wind, the lowest storage costs - up to just over £90. That's with the top storage costs, £45 a megawatt hour, 10% discount rate. So, somewhere in that range.
ML Let's just drill into one piece of that, which went past at speed, which was that you added £4 for transmission. But that's not for distribution, that's not for all of these homes that are going to be electrified. That's for, presumably, getting the electricity to where it's going to be electrolyzed to fill this long-term storage. Is that correct?
CL-S Correct. I mean it's £3 actually, £1 for batteries, £3 for transmission. So, we're calculating the cost of electricity that's at the power station gate, if you like, as it goes into the grid. The wholesale costs are somewhat different, because there are transmission losses, transmission charges, so on and so forth. And then it gets distributed, and that's totally different. But this is close to the wholesale cost, because there are two effects there, the inefficiencies and transmission charges. But we do have to say, what will it cost to get it from, you know, a wind farm out in the North Sea, to some salt-cavern in Yorkshire, or somewhere or other. So, we have to put in so many pounds per mile per megawatt for transmission. That's an interesting thing, actually: everybody agrees we're gonna have to invest a lot in the grid between now and 2050. But the question is, will that make transmission more expensive or less expensive? And that's not obvious, because we've made a bigger grid, but it's transmitting more power. So, per megawatt hour, actually, our ministry in their wisdom thinks the cost will go down. But we didn't assume, that we've always been conservative.
ML Now £3 per megawatt hour, if we gross up these figures to the investment in generation, transmission storage... How many billions are we talking about if you do it, instead of on a per megawatt hour, but you know, real money, as it were, the actual total billions?
CL-S So, if you take the wind and solar that we'll need. I took BEIS' last estimates of the cost of building, and I worked out how much more we'd have to build, that would have to get up to about 300 gigawatts. It's a mixture of solar and wind, by the way, because the wind is there in the winter, the solar is there in the summer. And if you mix them in the right way, on average, they can look a little bit like electricity demand, which is higher in the winter. Only on average; it fluctuates like hell from year to year. Anyway, if I take that figure, it's of order £100 billion in wind and solar. So, it's a lot, but not impossible. In storage, in the sort of base case of our storage - so this is for the store, for the electrolyzers that make hydrogen, and for whatever turns the hydrogen to power - it also comes out around £100 billion. Now, when I look at the grid, we have not studied the grid, but I have looked at the National Grid. And they say between now and 2050, we're gonna have to put £100 billion in the grid. So, this is only a small part of the extra transmission. So, there's going to have to be large investments, that's for sure.
ML To what extent is the hydrogen that you're going to be putting in these stores, to what extent could you characterize that as surplus? Using surplus electricity at times when you don't need it to keep the lights on, and the taxis running and so on?
CL-S Entirely, I think because, that's our design; design a system [in] which we have wind and solar, it meets demand directly, and we have extra to make hydrogen, that's it. But...
ML Where I'm going with that is, will that extra be in the right place? Because there's a lot of concern about curtailment. The problem with curtailment is there's a reason for it, which is we've not got the grid. It's sitting... It's tremendously blowy out in the North Sea, and how are we going to electrolyze somewhere near those hydrogen stores?
CL-S That's not the issue. In order to minimize the cost, you've always got to generate more electricity than you would literally need. I mean, there's a minimum value where you just have enough electricity to store and meet demand. But then you're storing every damn bit of wind, you have to have colossal power, you have to have huge stores, it bankrupts you. So, the case we looked at, with 570 terawatt hours of demand a year, you can get a system that works with 703 terawatt hours of wind, but it would be hideously expensive. If you just go up 10% above that, the cost drops, and then we get this broad minimum. So, there will always be some leftover. And that's a very interesting question. We have a surplus, which comes about in two ways: some of the time the store's full. I mean, you don't make the store bigger than it has to be, so the store's full, you can't store anymore. Or, the wind and solar power is so high in some hours, it would be madness to try and store it, because you build something to capture it, which was not needed most of the time. So, there is a surplus, which has two components where it comes from. So, the question is: it's being generated, would it have any value? So, we've thought about it. There could be uses for electricity which are not in our model of demand, because they don't exist today. But if there's cheap spare electricity going, somebody will think of a way of using it. So, I'll give you one example. One example would be drying biomass. If we're going to have a lot of biomass, people will be chopping it down, and then they're putting it in big hangars, and blowing hot air through it. So, that's a wonderful demand, because it doesn't matter when you do it. You can turn it on and off whenever there's a surplus, so that would be a use of it; what it would be valued at I don't know. But then you start to think, I can make hydrogen for other purposes. But when you come into that, there are bigger fish to fry, because a lot of people think there's going to be a lot of need for other hydrogen - people argue about what it's going to be for. Now, it seems to me obvious that if you studied producing hydrogen for all purposes, for transport, for industrial heat, etc., and for storage together, there will be system benefits, especially if the other was flexible. And the cost of each individual part will be lower than studying them separately. Now, we don't know how to do that for two reasons: we don't know how much hydrogen come in; but secondly, we don't know the hourly demand on it. So, in a sense, we're an upper bound in that sense too. Surely we're not going to waste the spare electricity, and surely we are going to need other hydrogen. And if you've costed it all together, the thing will get cheaper - I don't know by how much.
ML Let me just probe though. So, when you've costed the hydrogen, the production, where are the electrolyzers? Because the hydrogen storage is in these salt caverns, there's certain areas of the UK. Are you putting the electrolyzers close to the storage? Are you transporting electricity? Are you transporting hydrogen? And how many hours of use of the hydrogen electrolyzers have you assumed? I mean, these are really key drivers of the economics.
CL-S So, the fact is that we are going to be in surplus about 60% of the year. So, we'll be using the electrolyzers quite a lot of the time. Not all, because sometimes the store will be full, [inaudible]. We looked at that, and we think that the best option is to put the electrolyzers next to the store. It's cheaper to transmit electricity than it is to transmit hydrogen. That's not entirely an obvious statement. Because you can look up the International Energy Agency will tell you, ah, transmitting hydrogen costs so much a tonne per kilometre. But you can't use those figures, because you've got to look at the capacity at peak. So, we actually went back and calculated the size of the pipe you needed, the cost per kilometre, put in the discard rate. But it's pretty clear that it's going to be cheaper to transmit electricity.
ML I'm smiling because I had - Episode 115 - Jorgo Chatzimarkakis, who's the head of Hydrogen Europe. He's basically the leading lobbyist - I called him Europe's hydrogen pusher - and we had this quite fierce discussion about transport. And if what you're trying to transport is electricity to an electrolyzer, then it's quite clear to me, as it is to you, that you would do it as.... If you've got a choice, either to electrolyze and transport the hydrogen, or transport the electricity, you transport the electricity. But we had this quite fierce discussion, and I argued that... He was arguing also that the hydrogen should be distributed out to homes, to petrol stations, and that having two distribution systems would be cheaper than just one, which I find mystifying.
CL-S I don't understand that, and I don't believe we'll be transmitting it to homes, to heat our homes myself. But there is an exception to this, which is, if you could use a refurbished gas pipe, it might be cheaper. So, for example, there's a lot of offshore wind arriving in Scotland. Now there are north-south gas transmission lines, which end up near where we will put the stores.
ML But these are high pressure transmission... This is not distribution lines, there are transmission lines, and I agree totally.
CL-S We're not interested in distributing hydrogen, we're interested in taking large amounts and making electricity out of it.
ML So, you've come to... Coming back to the numbers, you've got then somewhere between £52 and £90, and that's your upper bound. You can then flex that with some other bits and pieces to try and bring it down. But that's kind of worst case, then, is £90 per megawatt hour?
CL-S Yeah. I think the worst case; I really don't see how we'll be at £45 a megawatt. Oh, I should say of course, this is all in 2021 prices. If you can tell me what commodity prices will be in 2050... And it's not going to be that number, there's inflation in there.
ML Let me come back now to Tom Samson's claim on his episode, which was that he can do nuclear - also in 2022 prices - and his upper bound for his small modular reactor, which isn't very small; that's 470 megawatts electric Rolls Royce SMR, he was saying £70. Now, the way I was trying to persuade him that this might be used is, you might be electrolyzing hydrogen for, let's call it 40 weeks a year, and then for 10 weeks a year, when there's low wind, low solar, those times of correlation that you talked about, Chris, that you might get a very high electricity price on the power markets. And so, you'd stop electrolyzing, and flip over and serve the power market, just serve it into the grid. And so, you could be getting an average that meets his maximum; he was talking about £40 to £70 for his output. So, isn't that a good role for nuclear?
CL-S Possibly, but I have not... As a matter of fact, it's the next thing I plan to do, I haven't modeled that seriously. But it's a complicated issue, because people tell you, if you use nuclear to make hydrogen, it's cheap, because you've got high temperature, and the electrolysis is more efficient. But if it's using up heat to make high temperature, it's making less electricity. So actually, the gain is not as big as people claim. And I actually think almost certainly that doesn't work. But I wouldn't go to the stake on that. However, there's one other thing we didn't mention, because you just said upper bound, again. I said that we did model using compressed air energy storage, together with hydrogen. So, compressed air energy storage, it's got to be what I call advanced compressed air energy storage. There have been systems around, running for 30 years or more, in which you pump up a lot of air to high pressure, you keep it in an underground cavern, you let it out again, and it turns a turbine. Now the problem is, as you know, if you've pumped up a bicycle, when you compress air, it gets hot. And the converse of that is when you expand it, it gets cold. So, these systems freeze. So, the systems that exist, burn gas to stop it freezing. Now, we can't do that in a net-zero world, if we want a lot of it anyway. So instead of that, what you have to do is when you compress the thing, you store the heat, and then when you expand it, you put the heat back again. So, as well as an underground store of air, you have a store of heat. And as a matter of fact, the heat stores most of the energy. The air stores what's called exergy; this is the ability to do work, which it does. So, we've modelled that. It's difficult because there are only two substantial systems of this type in the world in operation, and they're both 300 megawatt hours; we want gigawatt hour systems. So, we don't know how to cost it properly. But we've tried to do it anyway, and we think that for a reasonable range of assumptions and efficiencies, it would lower the cost, from the numbers £50 to £90 I was talking of, by possibly as much as 5%.
ML I'm feeling very, very good about my academic choices when I was 19 - so, we're talking 40 years ago - because I did go big on thermodynamics. And everything we're talking about is about moving around energy, moving around heat, storing heat, reproducing it, or reusing it, and then that fantastic word that you used, which is exergy. So, what you're saying, though, is that there are ways we can optimize, we can improve, and we can come down from your upper bound, using something like compressed air?
CL-S But quite interesting, there's a point I should make: if compressed air brings the cost down, you say why don't I do everything with compressed air? And the reason for that is very simple: if you don't turn its content over pretty damn fast, it's too expensive. So, you've got to have some hydrogen there, you've just got to - or ammonia, or synthetic fuels. So, you've got to have the hydrogen. But what's interesting is, when you have more than one type of store, there's a system... The question arises, how do you schedule their use? So, whatever time it is, if in the next hour, there's going to be a surplus, do I put it in hydrogen or in compressed air? And if there's a deficit, do I take the energy out of... which one do I take it out? It's never been studied. So, we've come up with our own way that we think minimizes the... close to minimizing the cost, but it's not necessarily ideal. And it's: you want to turn the compressed air over very quickly, because that lowers the demand on the hydrogen, and it's more efficient. But what's interesting is, we come up with systems... Whereas the compressed air store, in terms of the energy it can store, is small compared to the hydrogen store, but it's delivering more energy, because you're turning it over the whole time. So, that's why I said earlier, I don't like answering the question, how much storage do we need? Because once I get into two [inaudible] store, I have a small store turning over very fast, and a large one turning over slowly - or larger.
ML I would argue that what you need is clever market design, so that everybody who owns one of these assets will use it at the right time. One of our other guests, Yanis Varoufakis, would say no, nationalize it all! And have some state commissar deciding who does what, when, in vast detail.
CL-S There are two issues here, but there's a chapter in our forthcoming report on the market. And the first is that... I told you that the stored energy in the case I gave you was £80 a megawatt hour; that's just the storage. If I put in the cost of the energy that goes into that store, it's £160 a megawatt hour. So, I've got a mixture of stuff at £160 and at £35. Now, who the hell is going to build that stuff at £160? Which is used very seldom, but without which the system won't work? So, in the present market, no investor in their right mind will put money into something like that, it'd be far too risky. So, there's going to have to be some sort of guarantees. I mean, a government's got to say, we're going need this, so we will guarantee the cost. That's the first big market issue: how do you get the stuff built? Because the present market will reward short term storage; storing things for a few hours to reduce the peak, it's very well recompensed, people invest in that. But the second question is, if you've got several types of store; we, as theoretical physicist or ex-theoretical physicist, have designed a way of scheduling it, which is not necessarily the lowest cost - that'd be very hard to get - but the best we can do. But in order for that to work, you'd have to have collaboration between the generators, and the storage. They're different people; how's that going to work?
ML When you say collaboration, I mean... It feels to me like what you're saying is that there is an algorithm that will say, well, what is the optimum use of these resources? But then there needs to be a market design which replicates the behaviours that drive that algorithm, and not a suboptimal one?
CL-S That's right. So, the best we could come up with... We didn't go as far as renationalization, because, where's the money coming from? And secondly, you lose competition. So, what we've thought of instead - we just throw this out as an idea, this is a scientific report, a technical report - you could have a central agency - something like the Bank of England Monetary Policy Committee, except it would take actions - they have instructions to deliver this. And they will be responsible for buying and selling power. So, they'd say, right, Mr. Liebreich, you're generating wind and solar, we will buy it, and we will sell it to the store, we think it should go into, so a sort of buffer. They would also procure capacity. So, it'd be like renationalization, in a sense.
ML It's not a million miles from what National Grid is doing around balancing, and around some other things. I just want to... Just one observation, now that you've translated the £80 storage turns into £160, because it has to also buy the power, and then it's not efficient in the way it uses it, so it comes out at £160: it does feel to me like that question about nuclear is really critical; because I'm pretty sure that Tom would say, he doesn't mind selling pink hydrogen at a loss for some part of the year, if he can get £160 pounds for different parts of the year. And as long as that part is long enough, he's gonna be in the money.
CL-S Well, we've done the calculation and it's not right.
ML Okay. Well, I'd love to understand,
CL-S I am planning to look at that in more detail. I'm pretty sure that it doesn't work. [inaudible] about the technology of making hydrogen. You can also make hydrogen with high-temperature nuclear reactors, by splitting water. But that's Generation Four reactors; we can't even build the present ones. We'll never get to Generation Four...
ML I'm just conscious of time, and I want to make sure that we've touched on a couple of other things. One is, you're going to have to have, are you not, a lot of generation capacity to go back from all this hydrogen, back into electricity? How many gigawatts of generators are you going to need? They're going to be cycling on and off lots of times, are they not?
CL-S That's exactly why this is better than the alternatives - we haven't come to that. So, we assume. Well, in our model, the maximum demand is 98 gigawatts in 2050. So, we say, we'll make sure we have 100, so if maybe there's a surge, it's a nice round number. So, in all the numbers I gave you, I have assumed that whatever is turning hydrogen or compressed air back into power can produce 100 gigawatts; that's in the cost, okay? But the trouble is, now you can ask.... So, that's the killer, if I wanted to say instead of that, I will get gas for CCS and run it flexibly, for example. Now, the problem is, it's got to have 100 gigawatts, but it's only producing 50% of the power. So, it's working on a load factor - the maximum, what it actually has to produce, divided by what it could produce - of about 10%. So, it gets outrageously expensive. And flexing nuclear would be even worse. Nuclear only makes sense steady, or [inaudible] making hydrogen, that could make sense. But we put that in there. By the way, one of the things that's interesting is the question of turning hydrogen into power. The literature tends to say it's probably going to be done by fuel cells. But we actually think that four-stroke engines may be better, which is quite interesting.
ML 100 gigawatts of four-stroke engines?
CL-S We would not... You could do that, it'd be a lot of motors, though.
ML But when you said 100 gigawatts... If you assume that there are times when there is literally no wind, and no solar. So, you've said that the peak demand... So, it's going to be a freezing cold night, or evening, where people might be, I don't know, driving around charging some cars, with the heating on, and there's nothing coming out of the wind and solar, so it's all coming out of your hydrogen?
CL-S That's right. And so, of course, one of the difficulties is, we only have - and I confess something that's a little bit of a weakness in our report - we've got this model of one year of demand, okay? And, and it was based on the weather in 2018, as a matter of fact. So, it's not just absolutely uniform and mathematical. We simply repeat that 37 times, with years with different weather, which means actually, we're probably underestimating the maximum missing energy, the peak, because the times when there's no wind are the times when it's very cold. So, this means we can't model these things very accurately. In the one year we've got, the maximum demand is 90, almost 98 gigawatts. The maximum missing demand we find in that period is only 88. But on the other hand, there are times where there's 0.2 of a gigawatt of wind and solar - I mean, really nothing. And those could - with more than 37 years, if we correlated properly - they could coincide.
ML So, the 100 then combines the fact that you'll never have zero wind and solar, but equally it could be worse than that year that you've used as a model? But who is going to build those 10%? I guess this comes down to the policy question which says, right, you need to have a policy framework that builds the wind and solar; a policy framework that builds the transmission, which it is going to need - and personally, I think you're gonna need a lot more than the £3 per megawatt hour, because it's all in the wrong places for where these electrolyzers will be; and then you've got to build the electrolyzers; and then you've got to build your hydrogen salt caverns, which we've not talked about as much as we probably should; and then you've got to build this capacity to generate it back into the grid at the right points in the grid where it can go into distribution, which is probably some more transmission expenditure. We have got none of that policy in place right now, have we?
CL-S No, but I mean, just going back to the numbers, the reason we get £80 a megawatt hour, without the input power, is because there's a lot of generating power, there's a lot of electrolyzers and a lot of storage. So, it's back to the question, but it's the average that matters; that's only contributing 15%, so it gets diluted down. So, that's now back to the policy. How do we incentivize people to think in terms of the average cost? Not the individual cost?
ML The policy has to do a lot more than that. Because in a sense, the model has to say, well, the average cost, this is the best way to do it. But the policy has to say... it has to pay for the most expensive resource that's used the least amount of time for the whole thing to still work.
CL-S That's all built into the cost I gave you. Let's assume that...
ML Well, it's built into the average, but it doesn't answer the question of, how do you get one of my good friends with all the money to put their hand in their pocket?
CL-S That's correct. So, it's a market question, it's not a technical question like. Those are the correct average costs, with the cost of generating this huge amount of power, and so on and so forth. It's a matter of... there's going to have to be... Investing in storage is a tricky business, because it takes a long time to build this stuff; it can be bloody expensive to make all these salt caverns. And then you've got to take a judgement: what can I buy and sell power at in 10 years' time? And what changes of government policy will there be? So, it won't happen unless there are guarantees. But if we don't do it, we're going to pay a hell of a lot more for electricity. So, somebody's got to grasp this nettle and say, we will come up and make guarantees: you're going to get paid so much a megawatt hour if you build this stuff.
ML Luckily, we now have a Department for Energy Security and Net Zero, and I'm very excited to see how that energy security piece manifests itself in in policy. Just on those caverns, before I leave you: where are they? What are they? Have we got enough salt cavern space? Has all of this been properly thought through? Can you talk about the salt caverns, that piece of it?
CL-S Okay. This is an important point for other countries, because not every country, or every region, has the capacity to build salt caverns. So, in the UK, supposing just for a round figure, we needed 100 terawatt hours of hydrogen storage. In East Yorkshire, there is a capacity - I have this from the British Geological Survey - to build over 100 times that, simply in East Yorkshire. There's enough to do it all in Cheshire, there's enough to do it in Wessex, though it's not very well characterized. So, there are three regions, which between them, can produce over 100 times what we need. So, there's no question about the capacity. The way they work, these are called solution-mined salt caverns. So, you drill a hole down... And this has been studied in great detail, because something called the H21. North East Consortium, that's Scottish and Southern electric, and Equinor and some other companies, who wanted us all to heat our homes with hydrogen, but it doesn't matter, it's the same thing, they’ve costed it all. It's a 600-page report which we used. And then we say - we don't say don't believe it - we'll add 50% of the cost, just to be safe. So, the costs have that in it, the medium cost. So, they have these things about a mile underground. So, you dig down. The reason it's deep, by the way, is you want to be able to put high pressure on it. So, you've got to have a lot above it, or it'll blow the top of the thing. You drill down, and then you pump in water, it dissolves the salt, and then you pump out brine. And they have costed this and the BTS, British Geological Survey says, you could get enough of it.
ML And the reason that it needs to be so deep is that it's under pressure; so, if there are any cracks, you don't get leakage, what you get is the thing seals itself.
CL-S It would feel itself. It's more that you want... I think it's the pressure... You can't get to higher pressure than the pressure of the overbearing rock. By the way, one of the things with compressed air, of course, to store a certain amount of energy, you need about 20 times the volumes of hydrogen. So, that's one of the reasons you couldn't do everything with compressed air, you'd use up all this salt, you'd build every cavern you possibly can; that's not going to happen. But one of the difficulties is that most of the literature just assumes you can have any old parameters you want. But you can't, because there's a pressure range. So, you've got to decide what depth am I at, what pressure range am I at, how much energy, how much exergy, blah, blah, blah.
ML What you've presumably not looked at in this is that if we've got... you said it was 100 times as much in in East Yorkshire, then we've got Cheshire and we've got Wessex. Why would we not be doing this - storing hydrogen - for the whole of Europe? I mean, we've been blessed with this fantastic salt resource; as long as people are happy with this underneath their houses, which is a whole other conversation. Why would is not a fantastic export industry?
CL-S I have not thought about that. But there's quite a lot of salt capacity in Germany, actually. So, I doubt there's a case for it, and I think there's some in Spain as well. Somewhere in our report, there's a reference - though I haven't looked at it for a couple of years - where you find a map of the whole of Europe, and a map of the whole USA. I mean, I get asked, but surely this is completely new technology, how do I know what it will cost? Not true: there have been three large salt caverns of the sort of volume we want. Each of these caverns, and we need 90 clusters of 10 - they come in clusters because you share the surface facilities, that puts the cost down - we need 300,000 cubic meters. There are three such caverns in Texas, been operating for 30 years.
ML Caverns or clusters, can I ask?
CL-S Caverns.
ML So, we need 900 - 90 clusters of 10 - and the total global experience with this is three?
CL-S Five. There's a couple in Yorkshire. But I mean building... if you can build one, you can build two. But that's one of the reasons we put the cost up by 50%, because these things were constructed in the past. Although I'm sure Equinor did their best possible - and they ought to know about this - costing. I mean, I know a little bit from my experience at CERN about the difficulty of costing anything you do underground.
ML Very good. Look, there's so many great takeaways here. There's so many other directions we could take this conversation, and I hope that we get a chance to do that in person, I know that we're due to actually meet in a few weeks, and I'd love to do that. But the bottom line here is that it can be done: we can have a clean electric supply, that is of a scale that can decarbonize the whole of the UK economy. And the outside number that you've come up with is £90 per megawatt hour, which is, of course, expensive, compared to the £30, £40 pounds that we're used to in the historic past with polluting power, but it's not off-scale. And if we can bring it in for £50, £60, £70, then it's certainly a perfectly viable future.
CL-S Over the last year, in most of last year, the wholesale price of electricity was over £200.
ML Yes. But it was an exceptional year, we shouldn't take that as a desired destination.
CL-S We might have some other exceptional years! You're right, you can do it, and the alternatives in my opinion are more expensive. Just one qualification, if you don't mind, Michael: you said we could decarbonize the UK; this was only doing the electricity.
ML Well, but it was growing the electricity to expand into the areas of heating and transport.
CL-S That's right, if you expand it. It's quite true, that if we went to a bigger system, the cost per megawatt hour would stay the same.
ML But very good, look, it's a huge pleasure speaking with you about this piece of work. I look forward to its publication, I shall enjoy the commentary around that and I look forward to meeting up with you in person. Thanks very much for joining us today.
CL-S Thank you, Michael. It's been a pleasure.